Examining Microalgae as a Substitute to Soybean in the Production of Aquafeed
Published · Updated
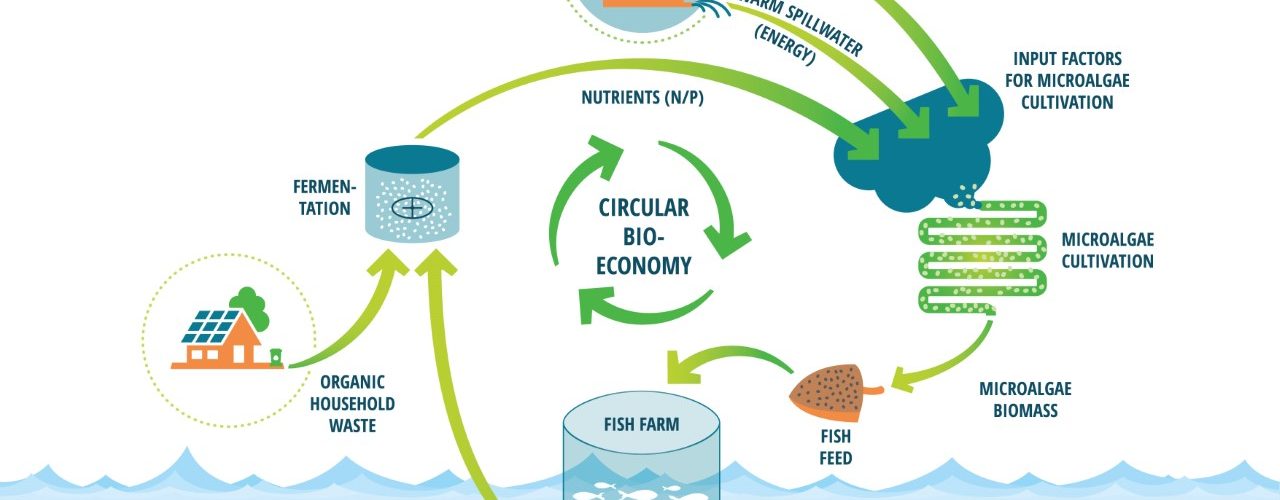
Et review av Hannah L.A. Gaustad*, Oda Bellika Kjæmpenes*, Paulina Pokusa*
* equal contribution
Introduction
Soybeans are a valuable resource for aquaculture because they can be used in aquafeed. However, some aspects of soybeans are problematic. Due to the issues associated with soybean cultivation and the final soybean-based product, microalgae could be a potential substitute for soybeans which is examined in the following review. Microalgae have several beneficial attributes which make them suitable for aquafeed, for example, balanced nutrition profiles and ease of cultivation. The following review focuses on the attributes of microalgae in aquafeed, microalgae cultivation, and the impact it would have if microalgae were applied in aquafeed.
Soybeans in Aquafeed
Soybeans are a versatile and valuable product in aquaculture, because of their nutritional and protein profiles that make them an ideal component of aquafeed (Park et al., 2017; Shea et al., 2020). Even so, there are two major obstacles that need to be accounted for when applying soybeans in feed – they are methionine deficient and contain trypsin inhibitor proteins. Methionine is an essential amino acid, making it a vital component of feed. If the concentrations of methionine are insufficient, the feed has to be supplemented with synthetic alternatives (Shea et al., 2020). The presence of trypsin inhibitor proteins is also detrimental because they inhibit trypsin, an enzyme that is important for protein metabolism (S.S., 2018). In order to overcome this issue, the product would need to undergo an additional processing step to render these inhibitors harmless (Shea et al., 2020). Other issues associated with soybean production are environmental, societal, ethical, and economic controversies, such as the questions raised about monoculture cultivation, slash-and-burn culture, uncertainty surrounding crop yield, and the industrial structured treatment of producers (Bicudo Da Silva et al., 2020; Edivaldo & Rosell, 2020; Miransari, 2016).
Despite these issues, soybeans remain a highly valuable source of aquafeed. In Norway, 800,000 tons of soybeans were used to produce aquafeed in 2017, which corresponded to 0,2% of the world’s total production (NHO, n.d.). This soybean-based feed also requires supplements from marine raw materials such as fish meal, which consists of ground-up fish (Hwang et al., 2014; NHO, n.d.). Whilst discussing this somewhat imperfect product, one might wonder whether there is a substitute where sustainability issues such as monoculture cultivation are avoidable. One possible alternative would be microalgae, which has been established as a strong contender for aquafeed within aquaculture. Not only would it replace finite resources such as fish meal and soybeans, but according to Ingmar Høgøy, the CEO and Chairman of the Board of AlgaePro, it could actually increase the quality of feed (personal communication, 29.10.2021). It has been demonstrated that microalgae-based feed provides several benefits, both animal welfare such as stress resistance and immunity, and consumer quality traits such as pigmentation increase as the fish were fed microalgae-based feed (Fernández et al., 2021).
Microalgae - a sustainable alternative to soybeans
Microalgae are unicellular organisms that can be either auto-, hetero-, or mixotrophic, which means that they obtain nutrition by their own means, through organic compounds, or both, respectively (Pereira et al., 2021). A subtype of autotrophic microalgae, the photoautotrophs, is the general focus of the following examination. Photoautotrophic microalgae convert inorganic materials, such as water, light, and carbon dioxide (CO2), into oxygen (O2) and glucose through photosynthesis. Water and CO2 are easily accessible because they are found in the environment where they are available for absorption. The light source for microalgae growth can be either artificial or solar; the solar light intensity varies depending on the time of day, weather, and season (Abu-Ghosh et al., 2016). The growth kinetics of photoautotrophic microalgae relies on the availability of carbon, which is mainly derived from CO2 (Hwang et al., 2014). In addition to inorganic compounds, macro-, and micronutrients, such as sodium, phosphorus, nitrogen, magnesium, calcium, and potassium, are also necessary for optimal microalgal growth (Khan et al., 2018). These might be sourced from fertilisers.
There are many types of microalgae, and several factors determine whether a strain is suitable for aquafeed, e.g., cultivation ease and nutritional content. Protein, vitamin, and unsaturated fatty acid content are especially important to determine the nutritional worth of microalgae (Colombo et al., 2020; Hemaiswarya et al., 2011). To fulfill the nutritional needs of aquafeed, it might be necessary to combine several algae strains because the vitamin profiles of one single algae strain might be insufficient. In addition to covering vitamin needs, the combined strains could balance out other nutritional compositions which would improve animal growth (Roy & Pal, 2015). Optimal fish development and growth rely on a balanced protein intake. The nutritional value of a protein source is considered high if it consists of amino acids that coincide with those that the feeding animal requires (Ammar et al., 2020; Roy & Pal, 2015). Fatty acid composition and concentration also appear to greatly impact the growth of the animal, and therefore, the lipid contents of the aquafeed biomass are vital (Patil et al., 2007). In addition, other factors, such as cultivation systems and growth, processing steps, risks, and potential improvements, should also be evaluated.
Microalgae cultivation and its challenges
Microalgae cultivation has the potential to grow into a powerful industry because it can be used to create a plethora of products. Some microalgae-based products include feed, medicine, pigments, and biogas fuel (Araújo et al., 2021). In order to fully grasp the potential of microalgae, cultivation means and challenges related to biomass production need to be assessed.
Cultivation of microalgae may take place in either open or closed systems. Determining which of these systems to employ is vital when it comes to biomass yield. There are both positive and negative aspects of either system, and the selection largely depends on external factors. The open and closed systems depend on natural light and the availability of the necessary technology. Internal factors, such as which strain of microalgae is grown, also affect this decision (Fernández et al., 2021). Open systems do not require as sophisticated technology as closed ones. However, they are more exposed to external factors such as precipitation, contamination, pH change, CO2, and O2 supply, temperature, nutrient availability, and light, which results in low system control (Fernández et al., 2021). Closed systems are more robust when it comes to these elements because they are not as exposed to their environment, and the parameters mentioned above can be rigorously regulated due to the complexity of these systems (Fernández et al., 2021; Ruiz Gonzalez et al., 2016). This rigorous control is the reason that the closed system is considered to be more efficient, as it is able to overcome challenges such as high temperatures or oversaturation of O2 (Ruiz Gonzalez et al., 2016). Yet, the yield of closed systems needs to be substantial enough to cover the building and operation costs, which means that it might be less sustainable than open ones (Fernández et al., 2021). Observations made in Spain have shown that the open system has a productivity level of around 27 tons ha-1year-1, while the productivity of the closed system is between 34-60 tons ha-1year-1, indicating that the closed systems result in a higher biomass yield (Ruiz Gonzalez et al., 2016). Closed systems are also beneficial when it comes to risk mitigation, as they have a lower risk of spillage. The consequences of spillages from large-scale facilities are elusive, and both the short- and long-term impacts of spillages need to be assessed. Some of the risks that need to be considered are whether the spillage will introduce invasive species, and how this will affect the surrounding ecosystem (Gressel et al., 2013). There are several means of risk mitigation that reduce the effects of a spillage, for example, a so-called safety switch in which the species that is being cultivated cannot survive in the surrounding environment (Asveld et al., 2019).
Another important fact to consider when selecting a system is which microalgae to grow, because different strains might require different growth conditions (Barkia et al., 2019). There are also major constraints that regulate the cultivation of certain microalgae. Administrative issues are one such constraint, and an example of this is the process required to cultivate species that have not been grown commercially yet. To get permission to grow these strains, there is a consulting process, in agreement with Novel Food regulation (EU), which is an expensive and long process. Another issue is the fact that microalgae vary greatly in their composition. This leads to a divergent potential for deriving functional compounds such as pigment, long fatty acids, antioxidants, and polysaccharides, and as a result, one microalga might not satisfy the end goal of the final product (Araújo et al., 2021). This issue could be overcome by mixing strains or genetically modifying a strain. However, both approaches can be problematic, and especially gene modification is highly regulated by international laws, such as “Regulation (EC) No 1829/2003 of the European Parliament and of the Council of 22 September 2003 on genetically modified food and feed” (European Union, 2003). Cultivation of genetically modified microalgae would also increase the risk that is associated with large-scale spillage, because the modified algae might spread and cause irreversible effects on local ecosystems, for example by out-competing native species (Beacham et al., 2017). To mitigate the effects that could occur due to spillage, genes that make the organism unfit for the surrounding habitat can be introduced alongside the desirable genes (Gressel et al., 2013).
A final issue that needs to be assessed is the harvesting step, which is considered to be one of the more costly steps (Pereira et al., 2021). Microalgal growth can be described as a cycle in which the growth has a lag, log, deceleration, stationary, and death phase. When introducing nutrition into the cultivation culture, the time duration of the lagging phase decreases. Hence, microalgae grow faster and obtain an optimum concentration, where it is at its most efficient point in the cycle. Once the optimal concentration is oversaturated, the growth rate decreases (Vasumathi et al., 2012). The goal of the harvesting step is to extract biomass from the culture medium to obtain as much high-value microalgal biomass as possible. There are several different techniques that allow the high-quality yield of biomass, such as electrical, chemical, biological, or physical. Still, there are disputes about which harvesting method is the most effective one (Mathimani & Mallick, 2018).
Applying food waste as a feedstock for microalgae is one addition that could make the industry more sustainable both economically and environmentally. Food waste has been shown to be a valuable option as a feedstock in microalgae cultivation, because of its rich and favourable nutrients, such as nitrogen, glucose, and phosphate (Pleissner et al., 2013). It is also a large and underused resource as there are about 88 million tons of food waste in the EU per year (approximately 173 kg per person), which represents about 20% of the food produced. Almost 70% of the food waste comes from households, food services, and retail stores, and the remaining percentage comes from the production and processing sectors (European Science Foundation, 2020; Sanches Lopez et al., 2020). If food waste was to be used as a feedstock, it could replace fertilisers, which are more expensive and less sustainable to use in large-scale microalgae cultivation (Usher et al., 2014). Cultivation on wastewater is also beneficial because organic wastewater from industries such as dairy and meat production cannot be added directly into freshwater sources due to eutrophication. However, this could be mitigated by using the wastewater in cultivation prior to releasing it (Ummalyma et al., 2022). This would promote a circular economy in which food waste collection and processing is connected to aquaculture, promoting industrial symbiosis (Figure 1). Yet, the application of food waste in microalgae cultivation can be challenging due to its collection, contamination, and processing. These can be overcome by developing a system in which food waste is carefully sourced from households and industries, to then be processed by fermentation or other processes that allows the waste to be circulated back into production processes. According to Toralf Igesund, who is the FoU leader from BIR, this would be more sustainable than disposal by combustion or deposition, because reusing food waste results in reduced emission and yields applicable products (personal communication, 05.11.2021).
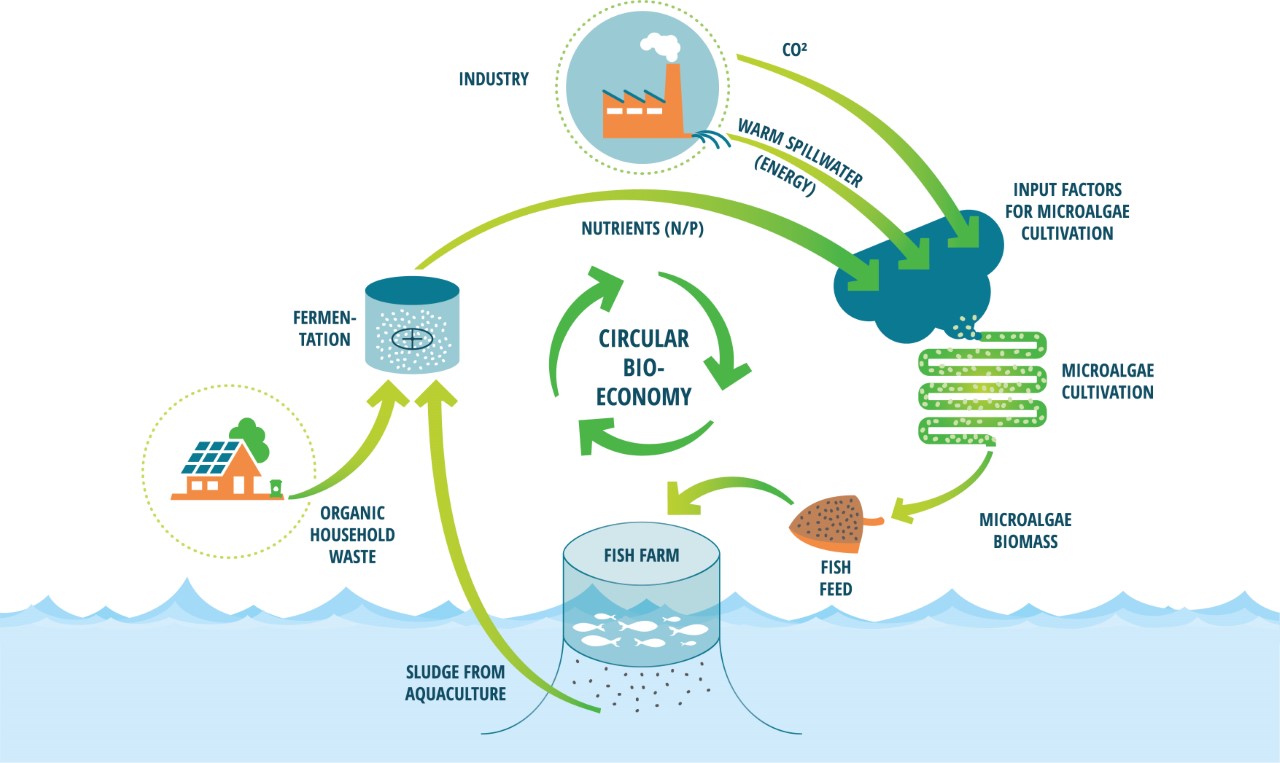
Figure 1 - Circular bioeconomy. The figure was provided and given permission to use from AlgaePro, 2022. Household waste and other sources of waste, as well as bi-products from other industries, can be funnelled into aquaculture with microalgae-based aquafeed as a steppingstone. This would be more sustainable and could also cut down costs.
In addition to funnelling food waste into cultivation, the cultivation of microalgae in general requires development in both economic and technical departments. Producing biomass is very expensive, due to both the resources and technology it requires, making it less economically sustainable (Pereira et al., 2021). The cultivation means are based on laboratory-scale studies, and up-scaling might be complicated, time-consuming, and could require government funding. Up-scaling will also necessitate stricter risk assessment because safety and environmental effects on a smaller scale might not coincide with the risks of a larger facility. The increase in risk might result in more stringent legislation that restricts cultivation facilities and the introduction of microalgae strains. In addition, the market for algae-based products needs to grow to make room for large-scale cultivation. Therefore, the future of cultivating microalgae depends on the government, researchers, and industries (Agency, 2020).
Environmental, societal, and economic impact
The impact of the research in algae cultivation affects not only the environment, but also society, economy, and science both in the long- and short-term, regionally as well as internationally. The growth of the algae industry can be seen in the context of the UNs Sustainable Development Goals (SDGs), and it will especially have an impact on the following goals: SDG number 9 “Industry, innovation, and infrastructure”, SDG number 12 “Responsible consumption and production” and SDG number 15 “Life on land” (FN Sambandet, 2021).
Environmental impact
Both positive and negative outcomes need to be examined when discussing the environmental effect of microalgae cultivation. One of the advantages of using microalgae is that they are capable of CO2 fixation by photosynthesis (Pereira et al., 2021). Other benefits include the fact that algae do not require freshwater to grow, as they can be grown in brackish waters, and water that is left over from industrial processes in both food cultivation and dairy production (Ummalyma et al., 2022). Using bi-products from industries as a resource promotes a circular economic stance, which can be more ecological because these bi-products are being reused to yield another product - this is a step toward reaching the UNs SDG number 12: “Responsible consumption and production” (Compass, n.d.). There are also negative aspects related to the use of CO2 in algae cultivation, which could potentially harm the environment. CO2 demand and the need for fertilisation drive up the ecological footprint of algae cultivation; this burden can be lightened by using wastewater or food waste as a fertiliser (Usher et al., 2014). However, there are several aspects that need to be considered when food waste or wastewater is applied for cultivation. One example is that the characteristics of the wastewater affect the choice of cultivation system, microalgae, and final appliances of biomass. It is also important to evaluate the source of the wastewater, where water from food industries is considered less hazardous than water from other industries as it does not contain toxic metals or other toxins (Ummalyma et al., 2022). An additional problem associated with CO2 demand is that it is not readily available as a substrate due to challenges related to dilution in water, as well as the uncertainty regarding how much CO2 microalgae actually fixate. Some articles state that algae's fixation of CO2 decreases the atmospheric concentration, whilst others argue that algae might release more CO2 than they absorb (Clarens et al., 2010; Pereira et al., 2021). These problems associated with large-scale cultivation suggest that cultivation might contribute to emissions at an unknown scale. However, some of these issues may be reduced by alterations made in production protocols and by applying regulations that mitigate the chance of these mishaps.
One subgoal of SDG number 12 is to reduce food waste per capita by half and to reduce food loss during production by 2030. By funnelling food waste into microalgae production, the amount of unprocessed waste can be sustainably lowered (Compass, n.d.). According to Toralf Igesund, the application of food waste in industries would decrease pollution related to waste disposal, such as methane gas from deposition or gases released during combustion (personal communication, 05.11.2021). In addition, it could also decrease the net amount of food waste that winds up in landfills (Pereira et al., 2021). Another advantage is that microalgae do not require fields to grow, meaning that they do not compete with food crops, terrestrial plants, or other native species. In contrast, other aquafeed sources such as soybeans require large fields of land which negatively affects biodiversity because native plants and insects might not thrive in these monocultures that are likely treated with pesticides (Wright et al., 2021). Since the microalgae industry competes and provides higher yields than terrestrial feed industries, it will likely impact “Life on land” (SDG number 15) because it might free up terrestrial areas.
Societal impact
Examining the societal effects of the microalgae industry can be better understood within the framework of how the aquaculture industry affects the labour market and smallholders. Development within the aquaculture industry is largely driven by commercial objectives, and when clusters of production and processing become prosperous, job opportunities may increase. This industrial growth also increases competition, which in turn may lead to higher product quality. However, it can also negatively affect smallholders because they cannot compete with large-scale producers. The fact that large corporate entities control the seafood industry negatively impacts small manufacturers which generate the best opportunities for rural communities (Belton et al., 2015; Little et al., 2016). Algae-based aquafeed would compete against other aquafeed sources, which could generate job opportunities within the algae industry but could decrease possibilities within other aquafeed industries such as soybean cultivation.
Since algae depend to some degree on the environment, the growth of industries will likely be local where enough water and sunlight are easily available. According to the EU Blue Bioeconomy report from 2019, this industry has great potential for the development of employment in coastal and remote areas (JRC, 2020). The decline of monocultures in this industry may also have a positive impact from a socioeconomic viewpoint. Since aquafeed production will not rely solely on soybeans, it might enhance the availability of products and raw materials.
The rise of microalgae cultivation would likely affect other niches than aquafeed, and products such as bio-oil and other algae-based products would likely be integrated into the current market. Applying food waste would also affect society by changing how waste is handled, both on an industrial and personal basis because traceability of waste funnelled into production is vital. According to Toralf Igesund, traceability from households can be challenging, but tracing waste delivered from larger food facilities is fully possible and can be done in accordance with the regulations of the Norwegian Food Safety Authority (personal communication, 05.11.2021).
Economic impact
Modernization and building new infrastructure for the future of microalgae cultivation also impacts the economy and is a step toward SDG number 9: “Industry, innovation and infrastructure”. The EU algae sector has an annual turnover of €1.5 billion as stated in the EU Blue Economy report of 2019 (JRC, 2020). According to Ingmar Høgøy, applying food waste in aquafeed production has an estimated turnover of 1.3 billion tons yearly, which highlights the potential for economic growth within this industry (personal communication, 29.10.2021). Another economic improvement is the fact that this industry, through its development within technology and competence, will stimulate other industries. If industrial symbiosis and regional clustering are achieved, economic strength would increase because it would allow several industries, such as food waste management, aquafeed, and aquaculture to grow together, and costs included in storage and transportation could be reduced. According to Toralf Igesund, establishing facilities close to each other could stimulate a circular bioeconomy, which again would strengthen the region where these industries are being settled (personal communication, 05.11.2021). This might make production more efficient and sustainable, as well as open the labour market regionally.
Summary
All things considered, microalgae show potential as a source of aquafeed and are likely to gain a stronger foothold in the aquaculture industry as it develops. However, it is important to keep the risks and regulations of microalgae cultivation in mind since it will likely impact how this field develops. The evolving industry of microalgae production might cause challenges for industries that normally filled niches such as soybean-based aquafeed production. Nevertheless, the foothold of these enterprises may be strong enough to withstand the competition which might cause stimulation of the industries to provide better products.
Hvordan referere
Gaustad, H. L. A., Kjæmpenes, O. B. and Pokusa, P. (2023). Examining Microalgae as a Substitute to Soybean in the Production of Aquafeed. Bikuben 2.
Referanser
Abu-Ghosh, S., Fixler, D., Dubinsky, Z., & Iluz, D. (2016). Flashing light in microalgae biotechnology. Bioresour Technol, 203, 357-363. https://doi.org/10.1016/j.biortech.2015.12.057
Agency, E. E. (2020). Bio-waste in Europe — turning challenges into opportunities. EEA. https://www.eea.europa.eu/publications/bio-waste-in-europe
Ammar, E. M., Arora, N., & Philippidis, G. P. (2020). The Prospects of Agricultural and Food Residue Hydrolysates for Sustainable Production of Algal Products. Energies (Basel), 13(23), 6427. https://doi.org/10.3390/en13236427
Araújo, R., Vázquez Calderón, F., Sánchez López, J., Azevedo, I. C., Bruhn, A., Fluch, S., Garcia Tasende, M., Ghaderiardakani, F., Ilmjärv, T., Laurans, M., Mac Monagail, M., Mangini, S., Peteiro, C., Rebours, C., Stefansson, T., & Ullmann, J. (2021). Current Status of the Algae Production Industry in Europe: An Emerging Sector of the Blue Bioeconomy. Frontiers in Marine Science, 7. https://doi.org/10.3389/fmars.2020.626389
Asveld, L., Osseweijer, P., & Posada, J. A. (2019). Societal and ethical issues in Industrial Biotechnology. In Sustainability and Life Cycle Assessment in Industrial Biotechnology (Vol. 173). Springer https://doi.org/10.1007/10_2019_100
Barkia, I., Saari, N., & Manning, S. R. (2019). Microalgae for High-Value Products Towards Human Health and Nutrition. Mar Drugs, 17(5), 304. https://doi.org/10.3390/md17050304
Beacham, T. A., Sweet, J. B., & Allen, M. J. (2017). Large scale cultivation of genetically modified microalgae: A new era for environmental risk assessment. Algal research (Amsterdam), 25, 90-100. https://doi.org/10.1016/j.algal.2017.04.028
Belton, B., Ahmed, N., & Murshed-e-Jahan, K. (2015). Aquaculture, employment, poverty, food security and well-being in Bangladesh: A comparative study. CCGIAR Research Program on Aquatic Agricultural Systems
Bicudo Da Silva, R. F., Batistella, M., Moran, E., Celidonio, O. L. D. M., & Millington, J. D. A. (2020). The Soybean Trap: Challenges and Risks for Brazilian Producers. Frontiers in sustainable food systems, 4. https://doi.org/10.3389/fsufs.2020.00012
Clarens, A. F., Resurreccion, E. P., White, M. A., & Colosi, L. M. (2010). Environmental Life Cycle Comparison of Algae to Other Bioenergy Feedstocks. Environ. Sci. Technol, 44(5), 1813-1819. https://doi.org/10.1021/es902838n
Colombo, S. M., Foroutani, M. B., & Parrish, C. C. P. (2020). Fats and Oils in Aquafeed Formulations. In Oil and Fat products Edible Oil and Fat Products (6 ed.). https://doi.org/10.1002/047167849X.bio095
Compass, S. SDG 12: Ensure sustainable consumption and production patterns https://sdgcompass.org/wp-content/uploads/2016/04/Goal_12.pdf
Edivaldo, T., & Rosell, S. (2020). Slash-and-burn agriculture in southern Brazil: characteristics, food production and prospects. Scottish geographical journal, 136(1-4), 176-194. https://doi.org/10.1080/14702541.2020.1776893
European Science Foundation. (2020). EU Platform on Food Losses and Food Waste. European Commission https://ec.europa.eu/food/safety/food-waste/eu-actions-against-food-waste/eu-platform-food-losses-and-food-waste_en
Fernández, F. G. A., Reis, A., Wijffels, R. H., Barbosa, M., Verdelho, V., & Llamas, B. (2021). The role of microalgae in the bioeconomy. N Biotechnol, 61, 99-107. https://doi.org/10.1016/j.nbt.2020.11.011
FN Sambandet. (2021). UN Sustainability goals FN 2021 https://www.fn.no/om-fn/fns-baerekraftsmaal
Frothingham S. (2018). Trypsin function: A proteolytic enzyme vital for good health. https://www.healthline.com/health/trypsin-function
Gressel, J., van der Vlugt, C. J. B., & Bergmans, H. E. N. (2013). Environmental risks of large scale cultivation of microalgae: Mitigation of spills. Algal research (Amsterdam), 2(3), 286-298. https://doi.org/10.1016/j.algal.2013.04.002
Hemaiswarya, S., Raja, R., Ravi Kumar, R., Ganesan, V., & Anbazhagan, C. (2011). Microalgae: a sustainable feed source for aquaculture. World journal of microbiology & biotechnology, 27(8), 1737-1746. https://doi.org/10.1007/s11274-010-0632-z
Hwang, J.-H., Kim, H.-C., Choi, J.-A., Abou-Shanab, R. A. I., Dempsey, B. A., Regan, J. M., Kim, J. R., Song, H., Nam, I.-H., Kim, S.-N., Lee, W., Park, D., Kim, Y., Choi, J., Ji, M.-K., Jung, W., & Jeon, B.-H. (2014). Photoautotrophic hydrogen production by eukaryotic microalgae under aerobic conditions. Nat Commun, 5(1), 3234-3234. https://doi.org/10.1038/ncomms4234
JRC. (2020). Algae biomass production for the bioeconomy. E. s. Hub.
Khan, M. I., Shin, J. H., & Kim, J. D. (2018). The promising future of microalgae: current status, challenges, and optimization of a sustainable and renewable industry for biofuels, feed, and other products. Microb Cell Fact, 17(1), 36-36. https://doi.org/10.1186/s12934-018-0879-x
Little, D. C., Newton, R. W., & Beveridge, M. C. M. (2016). Aquaculture: a rapidly growing and significant source of sustainable food? Status, transitions and potential. Proc. Nutr. Soc, 75(3), 274-286. https://doi.org/10.1017/S0029665116000665
Mathimani, T., & Mallick, N. (2018). A comprehensive review on harvesting of microalgae for biodiesel – Key challenges and future directions. Renewable & sustainable energy reviews, 91, 1103-1120. https://doi.org/10.1016/j.rser.2018.04.083
Miransari, M. (2016). Strategies, challenges, and future perspectives for soybean production under stress. Abiotic and Biotic Stresses in Soybean Production. In Abiotic and Biotic stresses in Soybean Production (Vol. 1, pp. 285-309). Academic Press https://doi.org/https://doi.org/10.1016/B978-0-12-801536-0.00013-X
NHO. What is soy? : NHO Mat og drikke https://www.nhomd.no/politikk/en-barekraftig-mat--drikke--og-bionaring/hva-er-soya/
Park, H., Weier, S., Razvi, F., Peña, P. A., Sims, N. A., Lowell, J., Hungate, C., Kissinger, K., Key, G., Fraser, P., Napier, J. A., Cahoon, E. B., & Clemente, T. E. (2017). Towards the development of a sustainable soya bean‐based feedstock for aquaculture. Plant Biotechnol J, 15(2), 227-236. https://doi.org/10.1111/pbi.12608
Patil, V., Källqvist, T., Olsen, E., Vogt, G., & Gislerød, H. R. (2007). Fatty acid composition of 12 microalgae for possible use in aquaculture feed. Aquaculture international, 15(1), 1-9. https://doi.org/10.1007/s10499-006-9060-3
Pereira, I., Rangel, A., Chagas, B., Moura, B. d., Urbano, S., Sassi, R., Camara, F., & Castro, C. (2021). Microalgae Growth under Mixotrophic Condition Using Agro-Industrial Waste: A Review. In IntechOpen (Ed.), Biotechnological Applications of Biomass.
Pleissner, D., Lam, W. C., Sun, Z., & Lin, C. S. K. (2013). Food waste as nutrient source in heterotrophic microalgae cultivation. Bioresour Technol, 137, 139-146. https://doi.org/10.1016/j.biortech.2013.03.088
Roy, S. S., & Pal, R. (2015). Microalgae in Aquaculture: A Review with Special References to Nutritional Value and Fish Dietetics. Proceedings of the Zoological Society, 68(1), 1-8. https://doi.org/10.1007/s12595-013-0089-9
Ruiz Gonzalez, J., Olivieri, G., de Vree, J. H., Bosma, R., Willems, P., Reith, J. H., Eppink, M. H. M., Kleinegris, D. M. M., Wijffels, R. H., & Barbosa, M. J. (2016). Towards industrial products from microalgae. Energy & environmental science, 9(10), 3036-3043. https://doi.org/10.1039/c6ee01493c
Sanches lopez, J., Pathina Caldeira, C., De Laurentiis, V., Salsa, S., & Avraamides, M. (2020). Brief on food waste in the European Union. European Commission. https://joint-research-centre.ec.europa.eu/publications/brief-food-waste-european-union_en#publisher
Shea, Z., Singer, W. M., & Zhang, B. (2020). Soybean Production, Versatility, and Improvement https://doi.org/10.5772/intechopen.91778
Ummalyma, S. B., Sirohi, R., Udayan, A., Yadav, P., Raj, A., Sim, S. J., & Pandey, A. (2022). Sustainable microalgal biomass production in food industry wastewater for low-cost biorefinery products: a review. Phytochem Rev, 1-23. https://doi.org/10.1007/s11101-022-09814-3
Regulation (EC) No 1829/2003 of the European Parliament and of the Council of 22 September 2003 on genetically modified food and feed, (2003). https://eur-lex.europa.eu/legal-content/EN/ALL/?uri=CELEX%3A32003R1829
Usher, P. K., Ross, A. B., Camargo-Valero, M. A., Tomlin, A. S., & Gale, W. F. (2014). An overview of the potential environmental impacts of large-scale microalgae cultivation. Biofuels (London), 5(3), 331-349. https://doi.org/10.1080/17597269.2014.913925
Vasumathi, K. K., Premalatha, M., & Subramanian, P. (2012). Parameters influencing the design of photobioreactor for the growth of microalgae. Renewable & sustainable energy reviews, 16(7), 5443-5450. https://doi.org/10.1016/j.rser.2012.06.013
Wright, A. J., Mommer, L., Barry, K., & Ruijven, J. (2021). Stress gradients and biodiversity: monoculture vulnerability drives stronger biodiversity effects during drought years. Ecology, 102(1), 1-n/a. https://doi.org/10.1002/ecy.3193