Investigating the number and colour of photophores of Maurolicus muelleri in two Norwegian fjords
Published · Updated
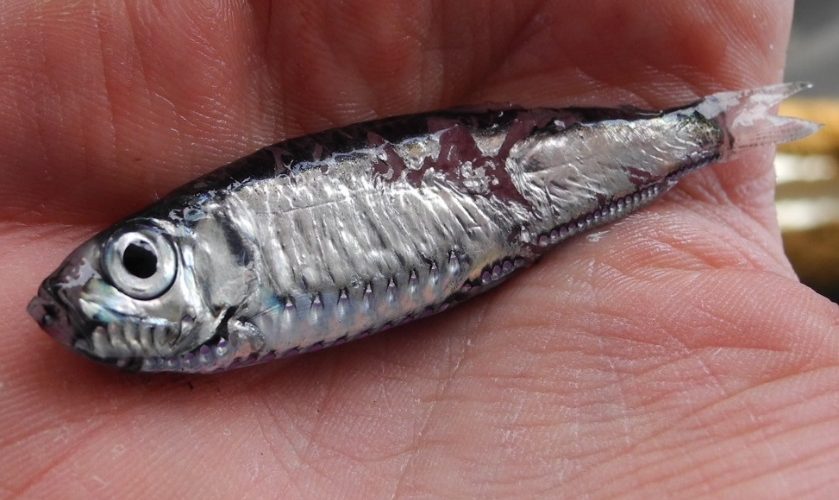
A report written by Anette Aune, Kristian R. Fjeld, Joseph C. Lawrence and Adam Mortensen
Abstract
Maurolicus muelleri is a dominating mesopelagic fish in Norwegian fjords, yet little is known about its photophores in relation to life history and environment. M. muelleri from Masfjorden and Fensfjorden were investigated to see whether body condition, age, environment, or depth have any effect on photophore number or colour. We found no relationship with body condition or age. However, our findings suggest M. muelleri higher in the water column (0-100m) have more photophores, and a higher proportion of them had green photophores than deeper down (200-300m). The observed proportion between pink and green photophores in total was 1:4. The total number of ventral photophores among individuals varied, with a mean of 87.9 ± 2.62, which is inconsistent from a constant amount of photophores previously reported in the literature. A higher proportion had pink photophores in Masfjorden than in Fensfjorden. Further research concerning the topic is necessary for a better understanding of this fascinating trait, and the next step seems to be investigating the relationship between photophores and light attenuation.
Introduction
The vast mesopelagic zone, often known as the twilight zone, is located underneath the sunlit euphotic zone and receives just enough light for vision (Christiansen et al., 2021). Irigoien et al. (2014) estimated the global biomass of mesopelagic fish to be between 6 000 – 200 000 million tons. This estimation is one order of magnitude larger than the earlier estimations, raising questions and interest about their global ecological importance in the world’s oceans, and the possibilities of potentially exploiting them as a novel marine resource. The exploitation of this resource would require more knowledge and understanding of both the ecology of these communities and the biology of the species involved.
The distribution of mesopelagic organisms in the water column depends on several abiotic factors (Aksnes et al., 2009). Many species, therefore, perform diel vertical migration, ascending to the surface at dusk and descending back into the depth at dawn (Staby et al., 2013). This allows mesopelagic organisms to stay undetected, whilst still staying at light levels which allows foraging, usually referred to as the antipredation window (Christiansen et al., 2021).
Life history is a term used for traits and strategies describing aspects of an organism’s life (Ratikaien, 2018). Examples of this are a species’ length, weight, sex, age, maturity stage, body condition, and eye size. It is pertinent to investigate the influence certain life history traits have over others, for example, how weight limits size, how sex can influence total length, and so on. These life history traits and strategies may even influence the development and function of specific organs (Stearns, 1992).
Maurolicus muelleri (Gmelin, 1789)
In the oceanic environment, the only light source apart from downwelling irradiance is bioluminescence, produced by marine organisms themselves (de Busserolles & Marshall, 2017). In fishes, bioluminescence is a common feature, with around 90% of fish exhibiting this trait (Herring, 1978). For many mesopelagic fishes it is used as a mechanism for communication, mimicry, attracting prey, and predator avoidance (Cavallaro et al., 2004).
Maurolicus muelleri is one species that use bioluminescence for counter illumination, hiding its silhouette from predators below. What defines this species are the distinct rows of photophores (bioluminescent organs) that run along the ventral side of the fish. Little is known concerning how life history strategies may control these organs’ development and function.
M. muelleri (Stomiiformes: Sternoptychidae), commonly known as Mueller’s pearlside, or simply pearlside, is a cosmopolitan fish species, and is one of the most abundant mesopelagic fishes in Norwegian fjords and the Northeast Atlantic Ocean (Grimaldo et al., 2020; Giske et al., 1990; Gjøsæter & Kawaguchi, 1980). They tend to be short lived, living no longer than 5 years, and typically grow to a size of 4-5 cm with a mean weight of 0.9g. (Giske et al., 1990; Folkvord et al., 2016). M. muelleri plays a key role as both a planktivore and as prey for large fish, like saithe (Pollacius virens) and blue whiting (Micromesistius poutassou) (Giske et al., 1990).
Individuals of M. muelleri distribute according to size in the water column (Staby & Aksnes, 2011) and this has been attributed to the conspicuousness of individuals in different life stages. Fry and juveniles are often small, transparent, and occupy shallower depths (from surface to ~100m) of the water column, where they can avoid predators. It has been described that M. muelleri performs diel vertical migration (Staby et al., 2013) and has specially evolved eyes. In the place of cone-cells, or rod-cells, it has rod-like cone cells, a combination of both, which allow individuals to see extremely well in low light, used as a mechanism to migrate alongside larger fish, whilst still avoiding predators (de Busserolles et al., 2017).
Photophores & Bioluminescence
Many marine species produce light (bioluminescence) from specialised light organs called photophores. Photophores are complex organs, consisting of light cells (photocytes) that produce the light through a protein-enzyme reaction of luciferin and luciferase, in addition to a reflector, and a lens (Cavallaro et al., 2004). The reflector covers the inner layer of the photophore, reflecting light produced from the photocytes towards the lens. The lenses functions to concentrate the light emitted towards the opening of the photophore (Cavallaro et al., 2004). In addition, some mesopelagic fish can utilize a masking pigment to cover the lenses of their photophores, thus regulating colour and intensity of light emitted (Clarke, 1963).
The photophores of M. muelleri can make up 10% of an individual’s body mass (Cavallaro et al., 2004). This heavy investment of resources may indicate that the trait is an integral part of their development. However, the metabolic cost of photophore production is unknown (Folkvord et al., 2016), and if this is a heavy cost, the fish must optimise the amounts of photophores regarding other life history traits, such as reproductive effort and body growth.
Individuals of Maurolicus species have usually developed most of their photophores when between 5-20mm (Rodrigues-Ribeiro et al., 2022). Adult M. muelleri have consistently been observed to have a maximum of 138 photophores (Cavallaro et al., 2004). This consistent maximum may be genetically determined, but the development of photophores from larvae to adult is yet to be documented. Folkvord et al. (2016) noted a relation between the development of photophores and the nutritional state of larval M. muelleri as their slowest-growing cohort tested seemed to have the lowest amount of photophores. Similarly, photophore number and life history traits have not been explicitly compared in the Norwegian fjords, an area in which M. muelleri is very abundant. The species is isolated from other Maurolicus species in this area, and the effects of interspecies isolation has yet to be described, whether there is any.
Aims & Objectives
M. muelleri is currently targeted by Norwegian trial fisheries for fish meal and oil, to be used in the fish feed industry (Fjeld et al., 2023). As there is a lack of knowledge regarding the biology and ecology of the zone, synthesizing more information on the species living there is vital before further exploitation occurs, to ensure sustainability and precaution regarding this understudied environment (Hidalgo & Browman, 2019; Fjeld et al., 2023). Despite their global abundance and importance in oceanic food webs, M. muelleri is an under-studied species, and especially regarding their light organs. Therefore, it is important to cover these gaps in the knowledge, as developing knowledge of a species allows the establishment of effective fisheries.
In this study, M. muelleri are sampled from two well-studied western Norwegian fjords, Masfjorden and Fensfjorden. From previous research cruises to Masfjorden and Fensfjorden, there was an observed photophore colour difference between individuals, whereas some individuals had pink coloured photophores, whilst others were green. As of now, there is no clear explanation for why this occurs, or how frequent it is.
There are many aspects of the M. muelleri life history that should be studied, especially in relation to photophores. In this study we focus on how photophore number and colour change in relation to depth and the life history traits, namely body condition and age. In addition, we compare M. muelleri from both fjords to see whether there are any differences between them.
Our main hypothesis of this study is that the number of photophores will increase with age, until maturity, where a maximum number of photophores will be reached (138). In addition, individuals with good body conditions will afford to maximise their number of photophores. Conversely, we expected those with a worse body condition to have less, as a trade-off between photophore development and survival. Moreover, we expect deeper residing M. muelleri possess more photophores both due to their larger sizes and to better perform counter-illumination.
Materials & methods
Research Area
The study was carried out between 24–30 September 2022 in two fjords on the west coast of Norway: Masfjorden and Fensfjorden. Masfjorden is a 494m deep, 24km long arm of Fensfjorden, which at its longest point is 50km and 583m deep. These fjords are separated from each other by a 75m deep sill. Data was collected from both fjords to provide the possibility to compare the influence of environmental conditions on the photophores and M. muelleri itself. The vessel used for transportation and data collecting was G. O. Sars, a research vessel shared by the Institute of Marine Research and the University of Bergen.
Field Methods
Individuals of M. muelleri were collected using a pelagic trawl fitted with a multisampler, with three codends at fixed depths (sub-layers) of 300m-200m, 200m-100m, and 100m-0m with an oblique haul through each layer. We trawled for approximately 10 minutes per codend, with an average speed of 2.5-3 knots. The multisampler had a mesh opening of stretch 22mm. Two trawl hauls were done in each fjord and each trawl was done between 8pm and 4am. We took a semi-random subsample of maximum 34 individuals of M. muelleri from each codend. If individuals were damaged to the point where it would have been impossible to count the photophores, they were discarded, and new individuals were selected, based on whether counting the number of photophores appeared possible or not. When the total number of individuals was 34 or below, all individuals were taken. In addition, we collected a random sample of M. muelleri from each codend, to compare with our handpicked individuals showing whether they are representative of the total catch. The subsampled individuals were put on a laminated sheet and scanned with Canon CanoScan LiDE 400. The scans were measured in ImageJ, using standard length. We registered whether the fish had green or pink photophores, and individuals with at least 40% pink photophores were assigned ‘pink’. As individuals had several shades of pink, from pale pink to purple, we simplified their colour to only pink, reducing errors due to human subjectivity.
Lab Methods
In the lab, we defrosted and weighed each fish individually (wet weight) in its plastic bag and subtracted the average weight of the bags. We then registered the number of photophores for each section of the fish, following the protocol described by Sutton et al. 2020 (p. 68) for the first 25 individuals of each codend. In the case of individuals being very damaged, we discarded that fish and used one of the remaining 9 individuals. After counting photophores, we extracted sagittal otoliths to determine age. We determined the age by counting increments on the otoliths, starting at age 0, with no, or a very small increment and assuming formation of the rings begins at hatching and continues until the adult stage. Some fish over 1 year were described as 1+ (written Age 1.5), due to the outer winter ring being the same size as the outer summer ring. Age 2 had two clear winter rings. The data was processed in RStudio, R version 4.2.1 (2022-06-23). We used data collected from the same field course, conducted in previous years and in addition to the data collected this year on size distribution for M. muelleri, we checked whether our semi-random sample was a good representation of the population.
We made two models in R, one linear model describing the total number of photophores, with total number of ventral photophores as the response variable, and the fjord, depth, body condition and age as the predictor variables. The second model was a binomial model which used photophore colour as the response variable with the same predictor variables as the first model. Both models were made by finding the best fit through removing parameters in order of least significance.
An ANOVA was performed to check for significant relationships between the different parameters. This was repeated for other comparative analyses. Post hoc pairwise comparisons were also made to further investigate significance found in the ANOVA tests performed.
Body condition was calculated using Fulton’s body condition factor K (Fulton, 1904), (I)
where W is weight (g), and L is length (mm). 1000 is a scaling factor. This condition factor was used to compare against other measurable parameters, rather than estimating the body condition itself. In total, we weighed, registered colour, and counted photophores of 258 individuals, 138 from Fensfjorden and 120 from Masfjorden. 75 fish were not counted, only weighed and colour registered. During our analysis, we found that the number of photophores on the head was constant on all individuals. The only notable variation in photophore number came from the ventral section on the fish (PV, VAV, AC, as given by Sutton et al. 2020, p. 68). By only analysing these photophores, we can include a larger number of fish due to ignoring the instances where it was not possible to record the photophore number. In total, we have 192 individuals with counted ventral photophores, 120 from Fensfjorden and 72 from Masfjorden.
Results
The comparison between the length distribution of our data and the total length distribution of M. muelleri caught during night-time on the cruise showed our samples represented the total range of lengths.
Photophore Number
Only 124 of the counted individuals were in a condition where we could count all photophores. The mean number of total photophores for these individuals were 138 ± 2.61. Of all counted individuals, we were able to fully count the ventral photophores of 225 (Table 1). Of these, 92 where from 0-100m, 93 from 100-200m, and 40 from 200-300m depths. The mean number of ventral photophores for these fish were 87.9 ± 2.62.
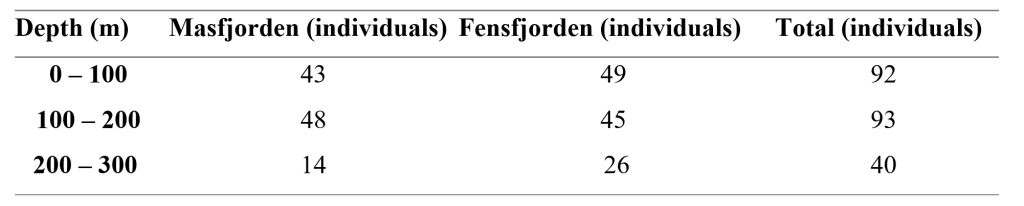
Table 1: Depth distribution of individuals of Maurolicus muelleri used in the analysis of numbers of photophores. Numbers from both fjords and total count are included.
Most individuals sampled in the study were of age class 1 (n = 202), with much fewer samples from age class 0 (n = 2), age class 1.5 (n = 15), and age class 2 (n = 6).
There was no significant variation in photophore number related to body condition (ANOVA; F(1, 221) = [0.83], p = 0.36), between the two fjords (ANOVA; F(1, 220) = [0.39], p = 0.53), or with age (ANOVA; F(2, 217) = [0.35], p = 0.412). During model selection, we found the only explanatory variable to be depth (p = 0.02), so further downstream analyses were performed using this best fitted model. We found a significant difference in number of photophores in relation to depth (ANOVA; F(2, 222) = [3.78], p = 0.02), with the mean number of ventral photophores of individuals at each depth being: 0-100m = 88.4 ± 2.67, 100-200m = 87.7 ± 2.50, 200-300m = 87.1 ± 2.59.
A post hoc pairwise test between total number of ventral photophores and individual codends showed no significant results between the depth layers 0-100m and 100-200m (Post hoc; t ratio = -1.85, estimate = -0.70, df = 222, p = 0.16), or 100-200m and 200-300m (Post hoc; t ratio = -1.16, estimate = -0.57, df = 222, p = 0.48). There was, however, a statistically significant result between the shallowest 0-100m and deepest 200-300m layer (Post hoc; t ratio = -2.59, estimate = -1.27, df = 222, p = 0.03).
In total, there was a trend of higher number of total ventral photophores in the shallowest layer, but no trends or relationships between age, body condition or fjord (Figure 1).
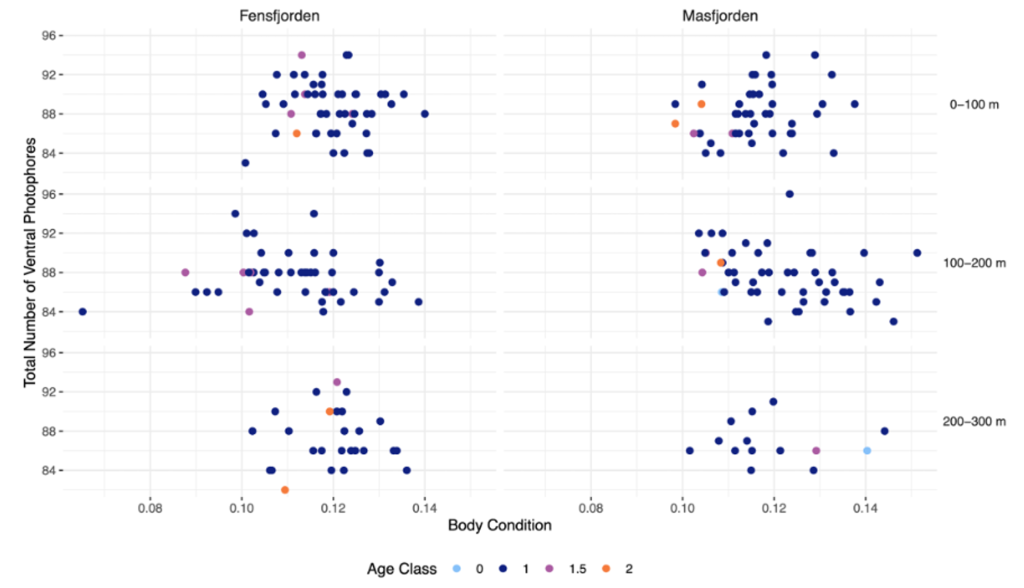
Figure 1: Number of ventral photophores in relation to body condition, depth, and fjord. The relationship between body condition and number of ventral photophores of Maurolicus muelleri in the two fjords Fensfjorden and Masfjorden. The depth at which the M. muelleri where caught are also included. The age of individuals is shown by the distinct colours of the points. Age was decided by counting otolith increments.
Photophore Colour
In total, we registered the colour of 289 individuals from all depth layers (Table 2). 217 of the individuals were green (75%) and 72 were pink (25%).
182 of the counted individuals came from Fensfjorden; 143 were green (78%), and 39 were pink (22%). The remaining 107 were from Masfjorden, of which 74 were green (69%) and 33 (31%) pink.
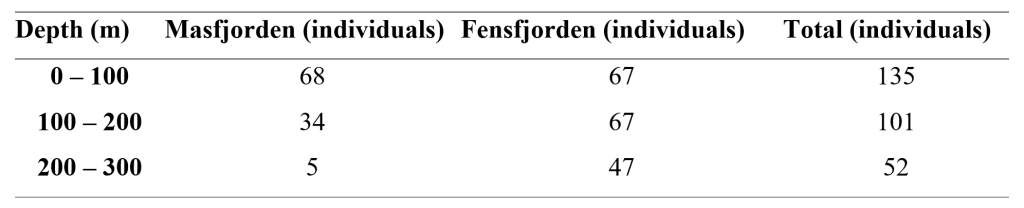
Table 2: Depth distribution of individuals of Maurolicus muelleri used in the colour analyses. Numbers from both fjords and total count are included.
Green coloured photophores occurred in higher numbers in both fjords and depths, except for the deepest depth where the proportion of pink is greater (Figure 2).
Using the best fitted model including only depth and fjord, a chi-squared test showed significant relationships between the colour distribution and fjord (χ2 (1, N = 289) = 16.67, p = <0.001), depth layers (χ2 (2, N = 289) = 44.50, p = <0.001). Age (χ2 (1, N = 289) = 2.11, p = <0.15) and body condition (χ2 (1, N = 289) =1.91, p = <0.17) showed no significant effect on the photophore colour.
A post hoc pairwise test between the observed colour of ventral photophores, and the depths sampled showed a significant difference in colour between the deepest (200m-300m) and middle layer (100m-200m) (Post hoc; p = <0.001 z ratio = 4.71, estimate = 1.92), and between the shallowest (0m-100m) and the deepest (Post hoc; p = <0.001, z ratio = 6.11, estimate = 2.73). There was, however, no significant difference between the shallowest and the middle layers (Post hoc; p = 0.06, z ratio = 2.24, estimate = 0.81).
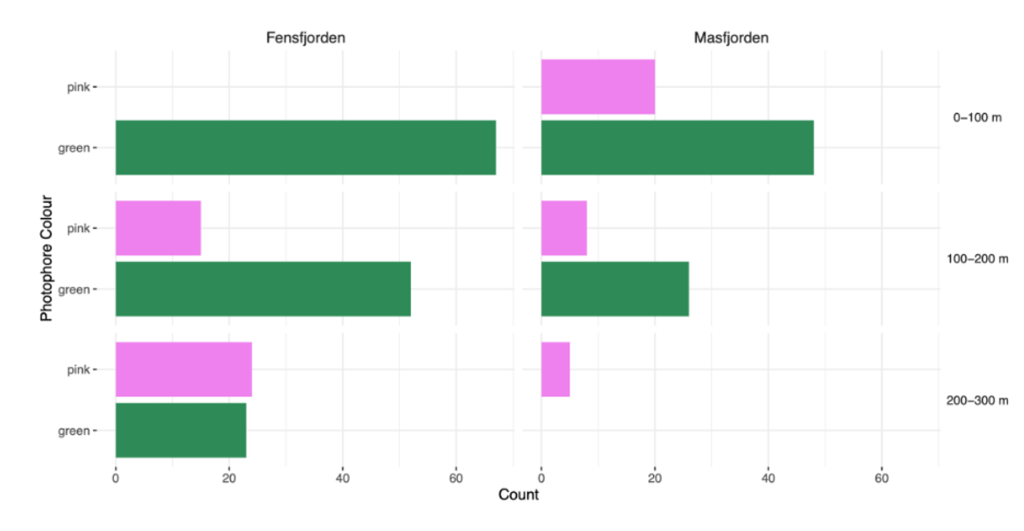
Figure 2: Count of photophore colour in relation to depth and fjord. The number of Maurolicus muelleri with green and pink photophores in the two fjords Fensfjorden and Masfjorden. The depth at which M. muelleri were caught is also included.
Discussion
This study is among the first on photophore colour and number, and how these varies in relation to aspect of the species’ life history. We found that M. muelleri at 0-100m depth have more photophores than those at 200-300m, yet there was much variation in the number at all depths. In total, green photophores was more common than pink, but the relationship between the colours changed between the depths. A higher proportion of pink photophores were found in the deepest layer compared to both shallower. In addition, Masfjorden contained a higher proportion pink photophores than Fensfjorden. In general, it looks like depth is the most crucial factor affecting both aspects of the photophores.
Photophore Number
It was interesting finding a higher number of photophores in the shallowest depth compared to the deepest. This was opposite from what we hypothesised, yet our hypothesis was based on knowledge of larger individuals preferring greater depths (Staby & Aksnes, 2011), which we did not find either. It could be explained by differences in light levels between these depths. The shallowest layer receives more light, and therefore M. muelleri here might need more photophores to produce sufficient light to hide its silhouette. However, no literature mention anything about such being found in any species. It might be a result of small sample size, especially for the deepest layer, as mean number of photophores per individual for each layer with standard deviations are quite similar and overlapping. This should be further tested to see if our pattern is indeed there or not.
The literature has consistently reported 138 photophores (Cavallaro et al., 2004), this is including a set of two photophores we did not count (ORB1 in Sutton et al. 2020, p. 68), meaning our mean is higher than the literatures’ in addition to showing more variation. Many species may use distinct photophore patterns in species recognition, and the number should therefore stay constant (Clarke, 1963). Davis et al. (2014) found that variation in photophore number within genera of Lanternfishes (Myctophidae) is not sufficient to be used in species recognition. Both findings suggest M. muelleri do not use number of photophore in species recognition.
The total number of ventral photophores of M. muelleri was not constant, having large variations (82 – 94). These variations are similar, although a bit larger than results of ventral photophore counts from the Red Sea (80 – 90) (Dalpadado & Gjøsaeter 1987). Moreover, we observed that some individuals had ventral photophores arranged asymmetrically, with one less photophore on one side. Further analysis to explain this asymmetry was not performed, as it is outside the scope of our project.
It has been postulated that the strict number of photophores is a mechanism of conspecific recognition. M. muelleri is the dominating species in these fjords, but also is the only species of the genus in these habitats (Giske et al., 1990; Rasmussen & Giske, 1994). It is very unlikely, if not impossible, they would encounter a species other than M. muelleri. This suggests that there would be no selection pressure to uphold a distinct amount of photophores, as in, if a neutral mutation entered these populations (if they are distinct populations), there would be no positive, and no negative effect if there is no need to discern between species.
However, since our counts from Norwegian fjords have comparable variation to counts from the Red Sea, it could indicate that variation in number of photophores of M. muelleri is a universal trait of the species, that can be observed in all the world’s ocean. For instance, if a constant number of photophores is inconsequential for counter-illumination functioning, variation in photophore number could arise from neutral mutations.
We observed significant difference in number of photophores between the shallowest and deepest depth layers. The hypothesis here was that individuals in the deeper layers would have more photophores, however, the opposite was observed, and that those in the shallower layers had more, and deeper had less. Following the idea that those deeper in the water column would need more photophores to visualize prey or communicate between conspecifics would not fit this as an explanation. A plausible reason for this observed trend could be that individuals living in shallower depths periodically encounter more predators. It has been described that the eye of this species allows it to exist amongst predators when performing diel vertical migration, having more photophores would perhaps allow them to better perform counter-illumination as a predator-avoidance technique, as such, those with more photophores are more likely to survive (Paitio et al., 2016; de Busserolles et al., 2017).
This could be another example of trait selection, although this case could be evidence for positive selection. Nothing more can be known about this unless genomic investigations are carried out. Similarly, following the trend identified in this study, these concepts do not fit with the established idea that the species distributes due to size (Rasmussen & Giske, 1994).
Photophore Colour
We have not found any literature concerning the colour of photophores of M. muelleri, meaning little is known about why we see different colours and what role it plays. In fact, there are pockets of research believing there to be only one colour, green. While it was impossible to comment on the cause of the colour difference, this study can state there were at least two colours in these specimens of M. muelleri, green and pink. An explanation for the pink colour could have been damage to the photophore structures, causing blood to stain the films. Another explanation is that M. muelleri may use masking pigments to regulate their emitted colour (Clarke, 1963).
On average, the ratio of pink individuals to green was 1:4. This is the first estimation of the occurrence of colour variation in photophores of M. muelleri. We do not know whether the colour is a static or dynamic trait. If M. muelleri can regulate photophore colour continuously, this estimation only provides a snapshot into how their colour varies during night-time in September, in these fjords. Potentially, pink and green could appear with different proportions throughout the time of day and could also vary with different seasons. In addition, we cannot know how photophore colour is affected by trawling, thus causing bias in our observations. A study conducted on live M. muelleri might be necessary to establish this.
Moreover, we observed that when photographing the photophores with flash on, the reflected light from pink photophores came out as blue, meanwhile reflected light from green photophores came out as green. This suggests that in nature, when light is produced from photocytes within a pink photophore, the visible colour emitted is blue, not pink. As blue light penetrates deeper into the depths than green light (Johnsen & Sosik, 2004), this finding makes us hypothesize that pink photophores are an adaptation to greater depths, making the M. muelleri better at hiding their silhouette as they better match the light conditions of their surroundings. Higher in the water column, more green light is present and green photophores would be preferable. The difference in photophore colour between the fjords could be related to this as well. Light attenuation of fjords increases when oxygen and salinity decline (Aksnes et al., 2009). If Masfjorden has less oxygen and salinity, we could assume it is darker at specific depths than Fensfjorden. If we had time and resources to investigate this as well, our colour hypothesis might have gotten a stronger validation.
Limitations
All trawling was done during night when M. muelleri from different layers are mixed. This could have affected the trends we found as it might not have been completely random which individuals migrated furthest and how they mixed. Repeating the study during daytime would test for this potential bias. Trawling in observed echo layers rather than fixed depths should provide data more representative for the layered vertical distribution of the species. In addition, our age data did not contain sufficient variation to investigate age properly and a relationship might still be found if this study was repeated with a larger sample of each age.
Sub-random sampling could bias our result, however our comparisons with total amount of M. muelleri caught during the field course showed our data was similar in distribution of sizes and covered the total length spectre caught. When counting photophores however, we should have been more critical, and discard all individuals with uncountable photophores to get a fuller dataset and to use total number of photophores instead of just ventral. The size of our sample should be larger, especially for colour from the deepest layer of Masfjorden.
We used Fulton’s factor as a measure of body condition even though M. muelleri seem to have a weight-length relationship parameter, b<3, while Fulton’s factor assumes isometric growth (b=3, Gubiani et al., 2020). This could result in over/underestimates of the condition of the smallest/largest individuals. Even though we found the log-relationship between weight and length to be ~3 it was lower, and another measure of body condition could be used to ensure our findings are valid and not a result of biased estimates.
Future Projects
To further investigate photophores and life history of M. muelleri this study should be repeated with larger samples and on a larger scale, allowing to compare populations in different ends of the species’ distribution. Measurements of light attenuation at different depths should be included to look further into the possibility that both number and colour are affected by the light level at the depth where the fish reside.
Sex determination of M. muelleri in our samples was not performed, so it is possible that number/colour of photophores have undiscovered correlations with sex. Species like ponyfishes (Leiognathidae) have been found to have differences between sexes in their bioluminescence organs (Sparks et al., 2005). The biochemistry of the photophores was also not covered in this study. Dissection and chemical analysis of differently coloured photophores could have helped uncover the mechanism and production behind the pink and green colours.
We noted during our study that the photophore colours were of varying intensities. Some had a strong pink/purple colour, while others had a lighter hue of pink. Similarly, some green photophores were strong and vibrant, others were dimmer. In our study, we assigned them as the categorical variables ‘green’ or ‘pink’. However, the colours could be explained better as a continuous gradient going from strong pink to bright green. We did not notice any pattern of different colour intensities with different depths, but this could be studied further. For example, through assigning colours as continuous variables through a digital program capable of registering colour and light emission from photophore pictures, rather than colour determination based on human vision.
Photophore number varied independently of body condition and environment (fjord), which is not expected for a costly trait. If the number are genetically determined, this could explain why. Based on findings by Davis et al. (2014), photophore number and patterns seem to be closely related to genetics in several species. At least from a genetic viewpoint and the data presented in this research, it is logical to assume many of these observations could be explained by neutral mutations present in these fjord populations of M. muelleri. Firstly, by defining populations between the open ocean, and even within the fjords, could be an effective way to investigate whether there is a distinct change in photophore number between different community assemblages (mixed with other Maurolicus species, or prolonged isolation as in the fjords).
Biogeography investigations could be used to identify for how long these individuals have been isolated within the fjords, or even if they have ever exchanged with open ocean populations. These isolated populations, and the differences observed from the standard, could even suggest a drift towards subspeciation, but for now at least it seems their isolation from other Maurolicus species has allowed an accruement of neutral mutations, that can be afforded. Using a retrospective model such as coalescent theory could identify when these mutations arose and give some context to the ecological influence and presence of these traits. Especially concerning photophore colour, investigating whether differences are observed in the individuals living with other Maurolicus species in the open ocean.
Authorship Contribution Statement
All the authors listed confirm that they have participated sufficiently in the work to take public responsibility for the content, including participation in the concept, design, analysis, writing, or revision of the manuscript.
How to cite
Aune, A., Fjeld, K. R., Lawrence, J. C., & Mortensen, A. H. (2023). Investigating the number and colour of photophores of Maurolicus muelleri in two Norwegian fjords. Bikuben 2.
References
Aksnes, D., Dupont, N., Staby, A., Fiksen, Ø., Kaartvedt, S. and Aure, J. (2009). Coastal water darkening and implications for mesopelagic regime shifts in Norwegian fjords. Marine Ecology Progress Series, 387, pp.39–49. doi:10.3354/meps08120.
de Busserolles, F. & Marshall, J. (2017) Seeing in the deep-sea: visual adaptations in lanternfishes. Philosophical Transactions of the Royal Society B: Biological Sciences. 372: 20160070.
Cavallaro, M., Mammola, C.L. and Verdiglione, R. (2004). Structural and ultrastructural comparison of photophores of two species of deep-sea fishes: Argyropelecus hemigymnus and Maurolicus muelleri. Journal of Fish Biology. 64(6), pp.1552–1567. doi:10.1111/j.0022-1112.2004.00410.x.
Christiansen, S., Klevjer, T.A., Røstad, A., Aksnes, D.L. and Kaartvedt, S. (2021). Flexible behaviour in a mesopelagic fish (Maurolicus muelleri). ICES Journal of Marine Science, 78(5). doi:10.1093/icesjms/fsa.
Clarke, William D. 1963. Function of bioluminescence in mesopelagic organisms. Nature. 198: 1244-46.
Dalpadado, P., & Gjøsaeter, J. (1987). Observations on mesopelagic fish from the Red Sea. Marine Biology. 96(2), 173-183.
Davis, M.P., Holcroft, N.I., Wiley, E.O., Sparks, J.S. and Leo Smith, W. (2014). Species-specific bioluminescence facilitates speciation in the deep sea. Marine Biology. 161(5), pp.1139–1148. doi:10.1007/s00227-014-2406-x.
Fjeld, K., Tiller, R., Grimaldo, E., Grimsmo, L. & Standal, I. (2023) Mesopelagics–New gold rush or castle in the sky? Marine Policy. 147: 105359.
Folkvord, A., Gundersen, G., Albretsen, J., Asplin, L., Kaartvedt, S., & Giske, J. (2016) Impact of hatch date on early life growth and survival of Mueller’s pearlside (Maurolicus muelleri) larvae and life-history consequences. Canadian Journal of Fisheries and Aquatic Sciences. 73(2), 163-176.
Giske, J., Aksnes, D. L., Baliño, B. M., Kaartvedt, S., Lie, U., Nordeide, J. T., ... &Aadnesen, A. (1990) Vertical distribution and trophic interactions of zooplankton and fish in Masfjorden, Norway. Sarsia, 75(1), 65-81.
Gjøsæter, J., & Kawaguchi, K. (1980) A review of the world resources of mesopelagic fish. FAO Fish Tech Pap. 193: 151
Grimaldo, E., Grimsmo, L., Alvarez, P., Herrmann, B., Møen Tveit, G., Tiller, R., Slizyte, R., Aldanondo, N., Guldberg, T., Toldnes, B., Carvajal, A., Schei, M. & Selnes, M. (2020) Investigating the potential for a commercial fishery in the Northeast Atlantic utilizing mesopelagic species. ICES Journal of Marine Science. 77: 2541-56.
Gubiani, É.A., Ruaro, R., Ribeiro, V.R. & Fé, Ú.M.G. de S. (2020). Relative condition factor: Le Cren’s legacy for fisheries science. Acta Limnologica Brasiliensia, 32. doi:10.1590/s2179-975x13017.
Herring, P.J. (1978) Bioluminescence in action (Academic Press).
Hidalgo, M., & Browman, H. I. (2019). Developing the knowledge base needed to sustainably manage mesopelagic resources. ICES Journal of Marine Science. 76(3), 609-615.
Irigoien, X., Klevjer, T., Røstad, A., Martinez, U., Boyra, G., Acuña, J., Bode, A., Echevarria, F., Gonzalez-Gordillo, J., Hernandez-Leon, S., Agusti, S., Aksnes, D., Duarte, C. & Kaartvedt, S. (2014). Large mesopelagic fishes’ biomass and trophic efficiency in the open ocean. Nature communications, 5(3271). doi:10.1038/ncomms4271.
Johnsen, S. & Sosik, H. (2004). Shedding light on light in the ocean. Oceanus Magazine, 43(2), pp.1–5.
Paitio, J., Oba, Y. & Meyer-Rochow, V.B. (2016) Bioluminescent fishes and their eyes. Luminescence. DOI: 10.5772/65385
Rasmussen, O. I., & Giske, J. (1994). Life-history parameters and vertical distribution of Maurolicus muelleri in Masfjorden in summer. Marine Biology. 120(4), 649-664.
Ratikaien, I. (2018). Livshistorie. [online] Store norske leksikon. Available at: https://snl.no/livshistorie [Accessed 14 Sep. 2022].
Rodrigues-Ribeiro, M., Suzuki, K. & Martins, R.S. (2022) Early development of pearlside Maurolicus stehmanni off south-eastern Brazil. Journal of Fish Biology. 100(2), pp.519–531. doi:10.1111/jfb.14962.
Sparks, J.S., Dunlap, P.V. & Smith, W.L. (2005) Evolution and diversification of a sexually dimorphic luminescent system in ponyfishes (Teleostei: Leiognathidae), including diagnoses for two new genera. Cladistics. 21(4), pp.305–327. doi:10.1111/j.1096-0031.2005.00067.x.
Staby, A., & Aksnes. D. (2011) Follow the light—diurnal and seasonal variations in vertical distribution of the mesopelagic fish Maurolicus muelleri. Marine Ecology Progress Series, 422: 265-73.
Staby, A., Srisomwong, J. & Rosland, R. (2013) Variation in DVM behaviour of juvenile and adult pearlside (Maurolicus muelleri) linked to feeding strategies and related predation risk. Fisheries Oceanography. 22(2), pp.90–101. doi:10.1111/fog.12012.
Stearns, S. C. (1992). The evolution of life histories (Vol. 249, p. xii). Oxford: Oxford university press.
Sutton, T.T., Hulley, P.A., Wienerroither, R., Zaera-Perez, D. and J.R. Paxton. 2020. Identification guide to the mesopelagic fishes of the central and south east Atlantic Ocean. FAO Species Identification Guide for Fishery Purposes. Rome, FAO. doi.org/10.4060/cb0365en
About the authors
Hi! We are the “Fjord Bros”. A group of MSc marine biology students, but more importantly - a group of friends. The Fjord Bros consists of Anette Aune (ecology and ethology girl), Joe Lawrence (genetics guy), Kristian Fjeld (mesopelagic fan), and Adam Mortensen (fisheries man).
Our group was formed by random assignment during the BIO325 “Ocean Science” course in 2022, and as fate would have it, through our shared passion and curiosity for the oceans - especially the mesopelagic fjord ecosystems, we developed a strong bond and quickly chose to name ourselves the Fjord Bros.
Our paper all started with a couple of very simple questions. How does the number of light organs (photophores) change as the mesopelagic fish “pearlside” (Maurolicus muelleri) grows? And why do some individuals have pink light organs, as opposed to green? To answer this question, we spent a week aboard G.O. Sars in Masfjorden and Fensfjorden, working long shifts at the wet lab sampling hundreds of pearlsides caught by trawling.
Back on land, countless hours and late evenings were spent counting each individual fish in a microscope. Through thick and thin, we stood together, determined to find the answers. As a result, through all this hard work, you are now able to read our paper which we are very proud to present. We hope you enjoy it.
During our writing process, we had regular meetups at K1/K2, to feast on the waffles served by biORAKEL. We would like to extend our gratitude to the people working there, ensuring that we had a great environment to meet and work in.